Introduction
Extended reality (XR) and its subsets, virtual reality (VR), augmented reality (AR), and mixed reality (MR), have expanded their roles in academia as researchers continue to seek out emerging technologies to solve modern problems. In the realm of teaching and learning, instructors are turning to XR learning objects as potential improvements on traditional learning objects. In response to this interest, universities have grappled with deploying VR learning objects in spite of associated costs and complicated logistics (Kavanagh et al. 2017). At the University of Florida (UF), Marston Science Library (hereafter Library) created MADE@UF, a virtual reality development space run by library staff, with the vision of providing VR technology to all of campus. The Library’s mission for MADE@UF is two-fold: supporting student learning and development of virtual reality, and aiding faculty in identifying, developing, and implementing VR experiences in curriculum.
In maintaining and coordinating a VR development space, the Library collaborates with faculty across campus from various disciplines including English, medieval studies, astronomy, psychology, and tourism, to name a few. These collaborations can involve identifying existing VR experiences to deploy as well as creating VR experiences for specific courses. In summer 2019, faculty from the Department of Chemistry approached the Library with an idea for VR experience to be designed for an Accelerated General Chemistry course in fall 2019. The Library assembled its team of experts, consisting of the Engineering Education Librarian, who is also the director of MADE@UF, the Chemical Sciences Librarian, and the 3D and Emerging Technologies Manager. Each Library team member brought their individual expertise in learning theories and pedagogies, chemistry education, and virtual reality development, respectively, to consider how creating a virtual simulation would benefit teaching and learning in this Accelerated General Chemistry course.
Constructivist Pedagogy in Virtual Reality
Constructivism as a learning theory involves the learner constructing their knowledge based out of their experiences in which the learner is an active participant (Glasersfeld 2003). Most VR experiences discussed in scholarly literature are not built on constructivist pedagogy; rather, most practitioners focus on and research intrinsic factors, such as immersion, motivation, and enjoyment, as essential to using virtual reality applications in teaching and learning (Kavanagh et al. 2017). This initial oversight is understandable, as before establishing the pedagogy of a virtual reality experience, the most fundamental aspects of virtual reality must be established. However, early VR researchers reference the importance of presence, accommodation, and collaboration while advocating for VR as a framework for constructivism (Bricken 1990). Immersion, another intrinsic factor, is fundamental in creating a virtual reality experience that is compatible with constructivism, specifically that “immersion in a virtual world allows us to construct knowledge from direct experience, not from descriptions of experience” (Winn 1993). These fundamental aspects of a VR framework must emphasize the importance of establishing identity, presence, and collaboration within a virtual space, all of which would be self-evident in physical spaces; this would then allow the learner to experience conceptualization, construction, and dialogue, which are staples of constructivist pedagogy (Fowler 2015). These intrinsic factors guide the creation and implementation of VR learning environments as frameworks for pedagogies to build upon (O’Connor and Domingo 2017).
In addition to focusing on intrinsic factors, researchers are also attempting to categorize VR learning objects retroactively under various pedagogies and learning theories such as experiential learning, situated cognition, or constructivism (Johnston et al. 2018). Of all the pedagogies and learning theories used in VR, constructivism is the most referenced pedagogy to accompany virtual reality experiences in education (Kavanagh et al. 2017). Constructivism is not inherent to all virtual reality experiences as it requires more than VR can independently provide. Rather, constructivist VR experiences should aim to provide feedback that results in revision and restructuring of previous knowledge constructs (Aiello et al. 2012). The VR experience also needs to include active learning, a component of constructivism in which learners derive meaning from their sensory inputs, so learners can freely explore and manipulate their environment while receiving sensory feedback (Chen 2009). VR experiences using a constructivist approach can facilitate knowledge construction and reflection as well as social collaboration (Neale et al. 1999). A benefit of a constructivist approach in virtual reality is improving the learners’ perceived usefulness of the learning material, which is the most significant contributor to positive learner attitude (Huang and Liaw 2018). A constructivist approach to VR has also led to gains in knowledge, skills, and personal development in a VR learning environment (Bair 2013). Spatial visualization, an important factor in chemistry education, has proven malleable and positively impacted by VR designed with constructivism pedagogy (Samsudin et al. 2014).
Chemistry Education Background
The molecular properties and chemical reactivity of compounds rely heavily on the way molecules are arranged and oriented in three-dimensional space, which is referred to as the stereochemistry of a molecule (Brown et al. 2018). A fundamental skill for chemistry students is the development of spatial awareness at the molecular level: understanding the structural geometries and relative sizes of molecules, as well as how to mentally translate between different visual representations of molecules, is a prerequisite to understanding and predicting chemical phenomena (Oliver-Hoyo and Babilonia-Rosa 2017). Teaching students how to visualize molecules in space is one of the quintessential challenges in chemistry education, particularly because the nebulous nature of chemistry concepts can be difficult to make tangible. Because there is no way to directly observe a molecule or molecular interactions at the sub-nanometer scale, models are used to represent chemistry concepts in both chemistry education and practice. A particular stereochemistry concept introduced at the undergraduate level is the chirality, or handedness, of organic molecules. Chirality refers to the relationship between objects that are mirror images of one another but cannot be perfectly aligned (or “superimposed”) on top of each other (Brown et al. 2018). This property is visible in all everyday objects that aren’t perfectly symmetric, such as a person’s left and right hands, threaded screws, and headphone earbuds. At the molecular level, organic compounds have a chiral center at any carbon atom with four different groups attached to it. Recognizing chirality and systematically naming chiral molecules are particularly troublesome tasks for undergraduate students due in large part to the difficulty of mental 3D visualization required to “see” these properties (Ayorinde 1983; Beauchamp 1984).
Research has indicated that handling concrete and pseudo-concrete representations of molecules (tactile models and computer-generated graphics) improves students’ spatial understanding of molecular structures in comparison to abstract 2D representations (Ferk et al. 2003). Educators have deployed a variety of visualization tools to help students translate the 2D representations of compounds in the pages of their textbooks into visualized 3D objects, including handheld “ball-and-stick” modeling kits and computer-based modeling programs. Ball-and-stick models were first employed in the mid-nineteenth century (Matthew F. Schlecht 1998) and are still the most widely used method for 3D visualization in undergraduate chemistry curricula. However, these model kits make a number of assumptions about molecular structures that are not accurate, including that bond lengths and atom sizes are all uniform. Commercially available modeling kits vary widely and leave more advanced visualization nuances to the imagination of the students. Computer modeling programs have the ability to represent individual molecules more accurately in terms of bond lengths, bond angles, and atom sizes because they do not rely on fixed physical pieces that the user assembles. Most of these programs are streamlined for ease of use and there are many free and open source software options for students to access (Pirhadi, Sunseri, and Koes 2016), including the popular programs Avogadro, JMol, MolView, and Visual Molecular Dynamics. The largest drawback of computer graphic representations for student learning is that they are not tactile and are typically viewed on a computer screen. A comparison of the 2D structural drawings common in chemistry materials, 3D models built with ball-and-stick model kits, and pseudo-3D digital images generated by computer software are shown in Figure 1.

Now that the costs of developing XR learning objects and obtaining the equipment necessary for students to experience them are becoming more obtainable, chemistry educators are exploring the use of AR, MR, and VR in the classroom. A review on the use of XR in education highlighted that course content being presented in a novel and exciting way, the ability to physically interact with the media, and the direction of students’ attention to the important learning objectives were all positive factors in the success of XR lessons (Radu 2014). Some examples specific to the chemistry domain include laboratory experiments designed in game engines like Second Life (Pence, Williams, and Belford 2015), AR smartphone applications that allow molecules to jump off the pages of lecture notes as 3D structures (Borrel and Fourches 2017), molecule building and structure interactions with AR (Singhal et al. 2012), environmental chemistry fieldwork simulated through VR (Fung et al. 2019), and VR experiences involving interactive computational chemistry (Ferrell et al. 2019). For teaching students about stereochemistry and chirality, the power of VR to bridge the divide between the structural accuracy of computer modeling and the tactile advantage of ball-and-stick model kits seems promising.
Many chemistry-education protocols have proposed that using multiple model types is the most beneficial approach for teaching students who may learn in different ways (Dori and Barak 2001). While there is evidence that viewing instructors manipulate computer models on a screen does improve student understanding in large chemistry lecture courses (Springer 2014), allowing for students to directly manipulate the model themselves has been suggested as the ideal approach to implementing computer modeling whenever feasible (Wu and Shah 2004). Encouraging students to translate between 2D and 3D representations during a facilitated interaction with 3D models has also been suggested to improve students’ ability to reason with chemical formulae, as opposed to students using models on their own with no instructor intervention (Abraham, Varghese, and Tang 2010). Combining these constructivist and chemistry education pedagogical insights, we chose to design and implement a lesson on visualizing, handling, and naming chiral organic molecules using an in-house built VR experience. During this lesson, the following strategies were employed:
- Undergraduate chemistry students in the class were previously instructed on the concept of chirality in their lecture course and had been exposed to 2D representations of chiral molecules.
- Each student had the opportunity to individually participate in the VR experience.
- Students were able to freely handle, rotate, and superimpose the molecules in the 3D virtual space.
- Students in groups were asked to make observations and explain the chemical phenomena in the virtual experience.
Design and Implementation of the VR Learning Object
The VR chirality experience was designed for CHM 2047, a one-semester, accelerated undergraduate General Chemistry course designed for students with a strong high school chemistry background who are interested in moving into upper-level chemistry courses. The course met three times a week with two lecture periods and one discussion period. The faculty member led the weekly lectures and split the students into five groups for the weekly discussion periods; each of the discussion groups was led by a peer mentor, an undergraduate student who had recently completed CHM 2047 and finished at the top of the class. Chemistry doctoral students were also involved in the course as teaching assistants (TAs) and participated in some supervised instruction as well as oversaw the undergraduate peer mentors. For the discussion period related to chirality, the faculty member for CHM 2047 solicited the expertise of the Library team to incorporate a virtual reality learning object. The Library team created a virtual reality template for classes to use in an assignment that allows learners to interact with 3D molecular models using virtual tactility and physics. During this interaction, the Library team devised a constructivist approach for the learning object.
Learners would recall knowledge learned in prior and current chemistry courses, specifically knowledge related to chirality and systematically describing chiral geometries. Drawing on this knowledge, students in groups would hypothesize and discuss their observations of the virtual environment and the molecules within it. Students would interact with other group members, testing their ideas about the virtual experience, and constructing an understanding of the learning object. Ultimately the objective for the students is to locate the chiral center of a molecule, describe the geometry of this chiral center, and realize the non-superimposable nature of chiral pairs. Additionally, students may be able to create a mental visualization of the molecules and improve their spatial awareness.
In order to prepare the VR template for use in the course, the instructing professors were asked to compile a list of relevant chiral molecule examples, generate computer models of these molecules using the software of their choice, and provide the models to the Library team in .PBD file type form. Although this activity was focused on small organic molecules, the Library team proposed this workflow because .PBD file types can accommodate small molecules as well as large macromolecules, such as proteins and polymers. This practice would allow for the use of protein structures from the Protein Data Bank (PDB), a global archive of 3D structure data of biological macromolecules (wwPDB consortium 2019), in future VR activities with ease. It is also possible to allow students to directly generate structures and provide them to the Library team, rather than the course instructors, as a part of the chirality lesson. The Library team was then able to import these 3D models into the game engine while retaining all color information provided in the original software. The Library team used an in-browser file converter designed by chemists to rapidly generate XR files from chemical structure files called RealityConvert (Borrel and Fourches 2017) to process the models from their original filetype (.PDB) to .OBJ 3D models with associated .PNG and .MTL files for mapping color to the model’s topography.
The team chose Unreal Engine V 4.20 because it is free to use for educational purposes and boasts pre-built VR interactive tools. Aside from its practicality, Unreal Engine can reproduce a project for Windows, Mac, mobile, HTML5, and other platforms. Once the VR template is set, it is relatively easy to drag and drop a new molecule model into the program and view it in immersive VR. The template was designed to show every loaded model in a museum-style room on a pedestal with the name of the molecule displayed above. The learner can approach each model, walk around it, and see from every angle. They can pick it up using motion controls and rotate the model in their hands. They can also grab a model in each hand to freely move the models around and compare. Once released, the model snaps back to its original position. For increased usability, the team felt it was necessary to design a physics object that had a natural feel when the viewer grabbed the model and rotated it using their own wrist and controller movement; this is notable as the team removed any physics interaction created by overlapping objects as well as the game engine’s own preset “gravity.”
The team chose a very plain room to model, using rectangular topography so as not to distract the learner from the molecules placed throughout the space; ample lighting was generated to create a well-lit space to explore. Additional lights were added below each of the models to highlight the topography and heighten the sense of three-dimensionality. The experience allowed the learner to move around the room by two separate methods depending upon the configuration of the VR experience. Either the learner could physically move through the space if using a VR setup that allows for full-range motion tracking; or the learner could use a trigger on the hand controller to point to a specific point in the virtual space and “jump” to it when releasing the trigger. A simple text document was provided to explain the controls.
The pedestals in the room were arranged according to a grid with three pedestals in each row. The learning objective of the VR experience was for students to compare the two versions of chiral arrangement for each molecule selected by the instructor. Chiral molecules are systematically classified as either R (“Rectus,” right-handed) or S (“Sinister,” left-handed) configurations. In each row of three pedestals, the R and S versions of the molecule were placed on the far left and right sides of the row. On the center pedestal of each row, a side-by-side display of both R and S versions was shown for the students to view. Because students were expected to determine and assign R or S configuration to the molecules they viewed, the R and S structures were intentionally randomized in regard to their positions on the “left” or “right” side of the room so as not to indicate chiral configuration. For example, one molecule might be arranged as R, R and S, S in its row in the room, while another might be arranged as S, R and S, R.
It is worthy of note that because the 3D models were placed in the VR space as non-rigid bodies—meaning that the objects can clip through one another and occupy the same virtual space—students were able to experience the non-superimposability of chiral molecules in a unique way. The defining feature of chiral molecules is that they cannot be perfectly aligned on top of one another, and typically ball-and-stick models of the two versions are held side-by-side as closely as possible to demonstrate this property. However, in this VR environment, students were able to hold one version in the same space as the other version for each chiral pair and see that no matter how they manipulated the models, they could not align all atoms in a way that matched.
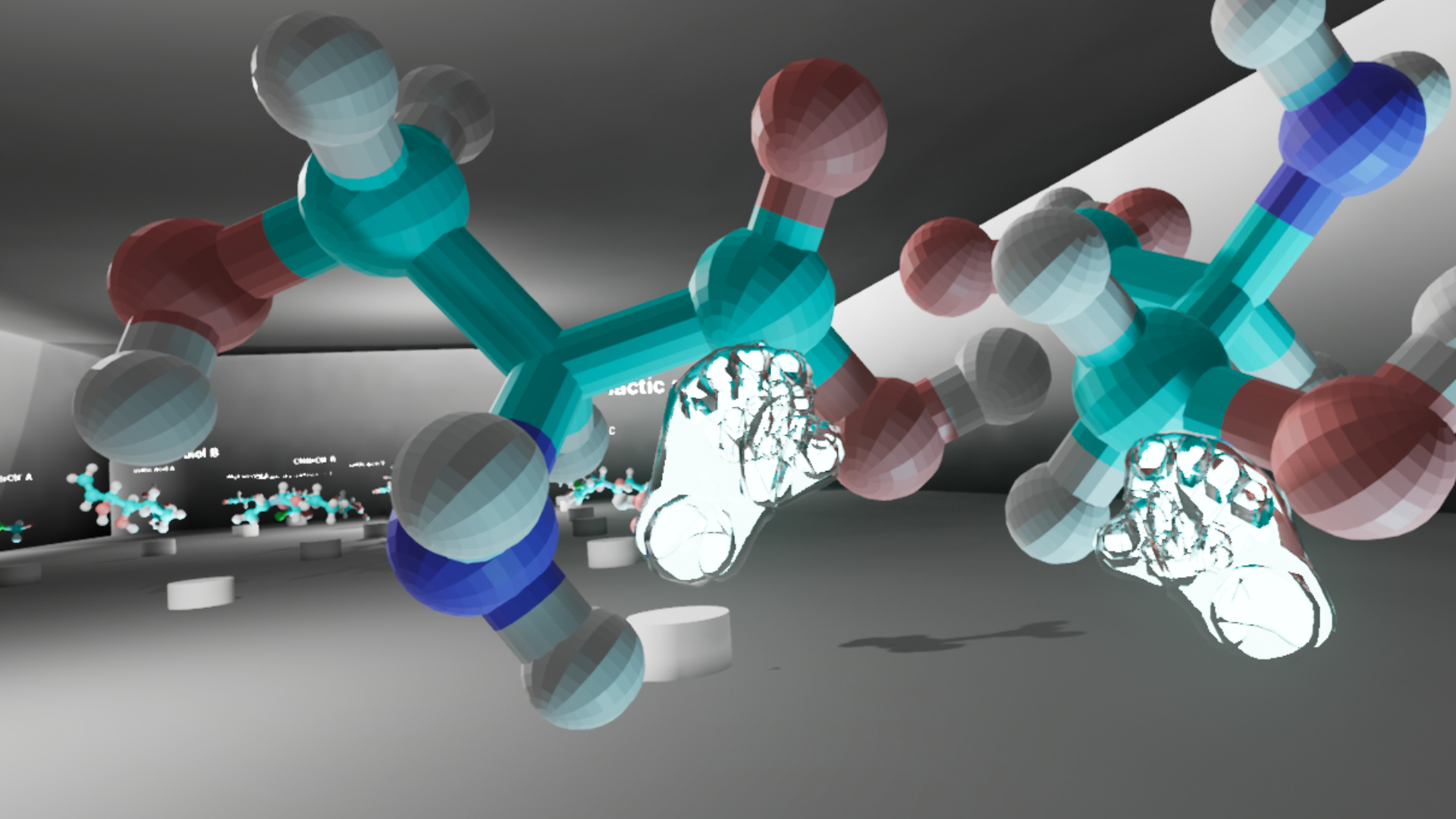
Once the template was updated to include the student-created models, the VR learning object was installed on VR-ready computers in the MADE@UF space at Marston Science Library. Library workers set up three Oculus Rifts on VR-ready computers in MADE@UF for five consecutive class periods on the day of a discussion period. Groups of two to four students moved to the VR stations, each with an Oculus Rift headset for the student and a monitor for the supervisor, a role filled by the peer mentor, teaching assistant, faculty member, or chemistry librarian. The role of the supervisor was to explain logistical questions with minimal input about the content of the experience, although supervisors would intervene if the students’ conclusions about the virtual experience were incorrect. The students interacted with the five sets of molecules, each set increasing in complexity as the student progressed through the virtual space. The students were able to manipulate, compare, and superimpose the two models in order to assign R/S configuration.
Further Use and Assessment
The CHM 2047 course instructor was looking to expose students to more advanced chemical concepts beyond the typical first-year general chemistry curricula in an innovative way. Chirality is a concept that may sometimes be introduced at the general chemistry level but is universally taught during the subsequent organic chemistry sequence. After the VR program was created and implemented in CHM 2047 during the fall of 2019, the same program was used for facilitated VR experiences in Fundamentals of Organic Chemistry (CHM 2200) and Organic Chemistry and Biochemistry 1 (CHM 3217) during the spring 2020 semester.
After these VR sessions were completed, a brief survey instrument (see Appendixes) was deployed in order to assess the student’s perceptions of the VR experience’s effectiveness in improving their understanding of chirality, including in comparison with other chemistry model types, and whether the students experienced any accessibility barriers during the process. Responses were collected from twenty-one students in total from the three chemistry courses.
Students were asked which molecular visualization methods they have used while studying chemistry and which they found most valuable to their understanding of chemical concepts. The four methods were VR (used by nineteen), ball-and-stick models (used by sixteen), drawings (used by nineteen), or a non-VR computer model (used by nine).

In terms of ranking how valuable each visualization method was, VR was ranked as the top choice with ten of twenty-one students, followed by drawings (seven students) and ball-and-stick models (four students). Two students ranked VR as their lowest choice method. Although nine students answered that they have used non-VR computer modeling before, none of the respondents ranked computer modeling as their top preferred visualization tool, which may be related to the intangible nature of computer modeling for novice chemistry learners.

The majority of students believed that virtual reality was a benefit to their spatial awareness of molecules. Eighteen of twenty-one students believed that manipulating the molecules in the virtual reality experience improved their ability to make R/S assignments. Sixteen of twenty-one students believed that manipulating the molecules in the virtual reality experience improved their understanding of the non-superimposibility of enantiomers. Lastly, seventeen of twenty-one students believed that manipulating the molecules in the virtual reality experience improved their ability to mentally visualize molecules. Students who answered in the affirmative to these questions often referenced that being able to see, visualize, move, hold, and touch the molecules was a benefit. One student described the experience as “incredibly helpful experience for someone like me that isn’t the best at spatial configurations,” while another mentioned that they “can still visualize how the molecules looked in the virtual reality experience and it has helped me to visualize molecules in my head.” A small group of students did not believe the VR experience was helpful. These students indicated that they already had an understanding of the concepts or that ball-and-stick models were superior. One student noted that “ball and stick models do the same without all the fancy equipment.”
The student responses to the survey highlight a need for improved methods for teaching content that requires spatial reasoning. While some students already have the requisite spatial reasoning skills, other students struggle with converting 2D, non-tangible drawings to a 3D mental construction. VR in chemistry can then serve as a tool to create more accessible content for a subset of students who historically have struggled with spatial reasoning. VR could then be used in conjunction with the traditional 2D drawings and ball-and-stick models.
One area of improvement for the VR experience was related to the visual accessibility of the program. Survey responses recorded that one out of the twenty-one respondents experienced “barriers” during the lesson, but this respondent did not disclose specific details of the accessibility issue. However, during one of the sessions hosted in the library, a library facilitator was needed to dictate the colors of specific atoms and indicate their identities to a user with color blindness. This accessibility concern is widespread in chemistry and chemistry education because periodic table elements are typically designated by a common color scheme and visualized molecules usually do not contain textures or patterns in addition to color coding. In future iterations of this VR experience, finding ways to depict atom identities that do not rely on color perception will increase user accessibility.
Reflection and Conclusion
Overall, the VR experience was successful. Chemistry faculty and TAs conducted informal debriefing sessions with the students following the Library VR session. Students provided positive feedback, with several noting an increased understanding of chirality following the VR experience. The faculty and instructors noticed the students were more engaged during the VR session than during other discussion or lecture periods, a feat that was observed to be uncommon for undergraduate chemistry courses. The course instructor mentioned that in previous years, the typical assignment on chirality involved students drawing 2D representations of 3D structures on paper; after the VR experience this semester, students commented on the ease of model manipulation the experience granted and said that they “truly understood” what the concept of chirality meant. The professor also noted that the undergraduate peer mentors (who had previously been students in the course before the VR lesson was implemented) “were particularly content on the new way to look at molecules, describing it as a more direct way to understand the role of 3D in chemistry.” The chemistry faculty are already interested in using the experience again for their Fall 2020 coursework, and several other chemistry faculty have also contacted the Library about deploying a similar VR learning object for their classes. The CHM 2047 professor commented that “it is clear from the success of this assignment that teaming up chemistry instructors with experienced librarians is the best combination to implement new technologies within the chemistry curricula.”